Beyond Discovery
High-resolution, super-precise instruments like NEID can do much more than just discover new exoplanets and measure their masses. For certain planets, NEID may be able to detect molecules in their atmospheres, or–as we will see here–measure detailed properties of their orbits. Today, we will look at the orbital properties of the young exoplanet TOI-2076b. This exciting result was led by NEID team member Robert Frazier, and is published in full detail in a recent publication in the Astrophysical Journal Letters.
Obliquity:
All stars spin on their axis, same as a spinning top or the Earth. The Earth, and most of the other planets in the Solar System, orbit around the Sun in the direction that it spins, but this is not always the case. They can actually go around the star in any direction, and their orbits can be inclined in any way. The angle between the axis on which a star spins (stellar spin axis) and the axis of a planet’s orbit around the star (planet orbital axis) is called the stellar obliquity, and is visualized here:
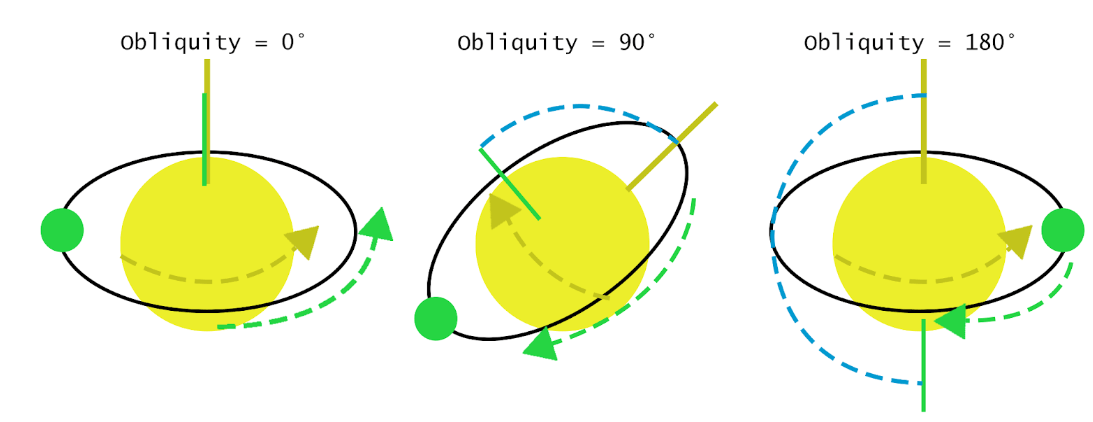
Figure 1: An array of possible obliquities are shown in this figure with the obliquity angle shown in blue. The stellar spin axis is shown in dark yellow and the planet orbital axis is shown in green.
As can be seen in Figure 1, there’s a wide range of possible obliquities and orbits. They vary from the planet orbiting around the star’s equator to orbiting above the star’s poles. Planets with a small obliquity are known as well-aligned, while planets with a large obliquity are known as misaligned.
Rossiter-McLaughlin effect:
Determining obliquity for stars and their exoplanets is difficult, requiring precise spectroscopic observations. It has only been done for around 200 exoplanets out of the over 5,000 confirmed exoplanets around almost 4,000 stars. Most of these have been determined using the Rossiter-McLaughlin (RM) effect. The RM effect occurs when a planet transits in front of its host star and blocks out light from the star as would be viewed by an observer. The RM effect is visualized in the following Figure:
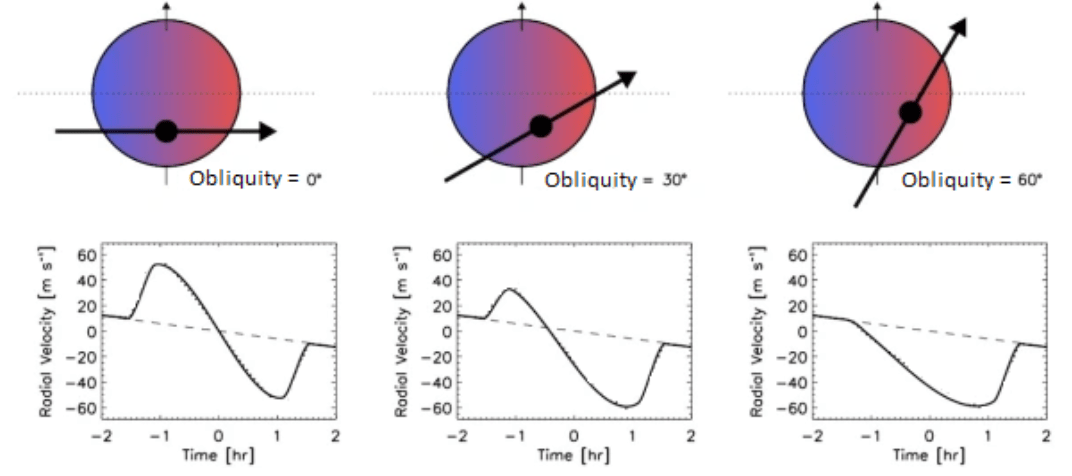
Figure 2: In this figure, three different transits of an exoplanet in front of its host star and the resulting obliquity and RM curves are shown. Due to the star rotating on its axis, it causes one hemisphere to be blueshifted, and the other redshifted as seen from an observer on Earth. The path of the exoplanet in front of the star is shown with the thick black arrows. The dashed curves show the expected RV signal if the planet was not blocking any light, while the solid line is the ‘RM curve’ showing the expected RV variations during the transit. The shape of the curve is highly dependent on the obliquity of the star. Figure credit: WASP-Planets.net
As highlighted in Figure 2, as the star rotates on its axis, the light coming from the side spinning towards the observer is blueshifted, while the light coming from the side spinning away from the observer is redshifted. Thus when a transiting exoplanet blocks a part of the star spinning towards the observer, it is measured as a net redshift as the blueshifted light is reduced, and vice versa. This creates the distinctive curve of the Rossiter-McLaughlin effect, and is used to determine stellar obliquity.
History of Orbits:
Early determinations of stellar obliquities found that they were mostly small, just as we would predict based on the obliquity of planets in the Solar System. Earth’s obliquity is only 7.2 degrees, making it fairly well-aligned. However, starting in 2008, many planets started to be found to have high stellar obliquities. The sudden change in these observations and the physical causes were not known, and it broke the idea that planets all orbit on well-aligned orbits. As the discoveries continued, it was found that among Hot Jupiters—planets the size of Jupiter that are very close to their host stars, and make up the majority of obliquity measurements due to their ease of detection—there was a split into two populations: well-aligned, and misaligned Hot Jupiters. This obliquity bifurcation means that a systematic mechanism (or mechanisms) had to be occurring, but it remains to be seen whether this bifurcation is intrinsic just to Hot Jupiters or a broader range of planets, including in particular smaller planets which are more numerous, but have remained more difficult to measure due to their smaller sizes.
NEID Observations:
We leveraged the exquisite RV precision enabled by NEID to measure the obliquity of an exciting recently discovered young Neptune-sized planet called TOI-2076 b. TOI-2076 b is a warm and young (200 million years old, compared to 5 billion years for the Solar system) sub-Neptune (2.4 Earth radii) that orbits around its host star, TOI-2076, along with planets TOI-2076 c and TOI-2076 d. It was first discovered by a research effort led by Christina Hedges in 2022 (see paper here) using observations from NASA’s TESS (Transiting Exoplanet Survey Satellite). Using NEID, we conducted radial velocity measurements of TOI-2076 and observed the Rossiter-Mclaughlin effect.
In a single transit with NEID, we were able to determine that TOI-2076 b is well aligned with an obliquity of -3 +/- 16 degrees, as can be seen in Figure 3. What was interesting to see was that there was an overall upward curve in the RVs, as opposed to the flat to slightly downwards curve normally expected with the RM effect. The RV curve can be seen here:
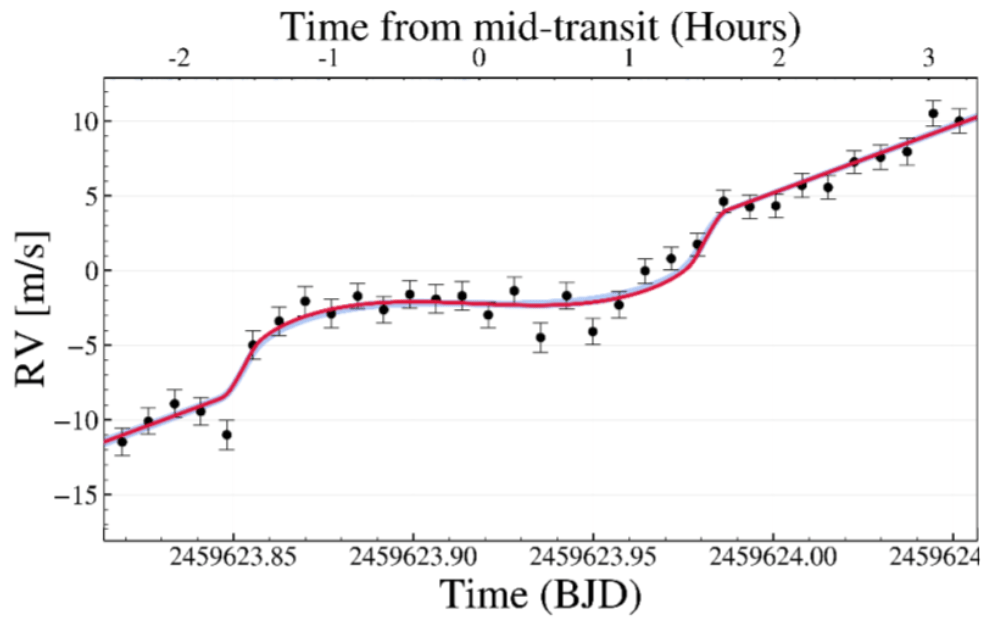
Figure 3: The RM curve captured by NEID. The RVs are plotted against the Barycentric Julian Date (bottom), and time from mid transit in hours (top). The black dots are the measured radial velocity values from NEID, the red curve is the calculated model for the RM curve based on the RVs, and the blue shading highlights the 1 sigma credible interval of the best-fit model.
We found that the curve of this slope changed depending on what range of wavelengths it was constructed from. Combining this with the star’s young age and clear brightness variations seen by TESS led us to attribute the overall upward trend in the RVs to starspots, regions of high magnetic activity analogous to Sun spots. Accounting for this slope, we had no difficulty in analyzing the RM effect and arriving at our measured obliquity.
With this measurement, TOI-2076 b joins a small but growing sample of young planets in multi-planet systems with well-aligned orbits, and is the fourth planet with an age less than about 300 million years in a multi-transiting system with an obliquity measurement. A plot of exoplanets in systems with multiple transiting planets with measured obliquities is shown below to put our measurement into context. Some values have been left out due to high uncertainty on the age or obliquity.
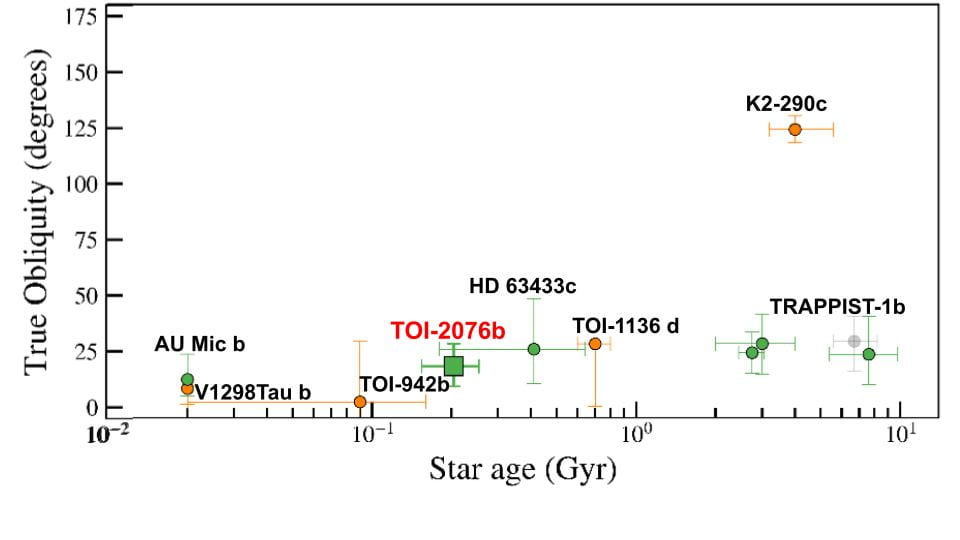
Figure 4: A plot of planets in multiple transiting systems with measured obliquities. TOI-2076b is shown as a square and is highlighted in red. The orange points represent Warm and Cold Jupiter exoplanets and the green points represent Sub-Saturn exoplanets. The grayed out marker represents a Hot Jupiter.
The low obliquity of TOI-2076 b, and the presence of transit timing variations in the system—which are variations in timings of transits of planets in a multi-planet system that are capable of gravitationally tugging each other to and fro—suggest the TOI-2076 system likely formed from an already well aligned protoplanetary disk. We believe that the two other observed exoplanets in the system, TOI 2076 c and d, are also likely to have well-aligned orbits, although additional observations would be required to confirm this prediction.
Lastly, TOI-2076 is also an exceptionally bright star, and so this provides the opportunity to characterize the atmospheres of all of the three transiting planets in the system with, for example, the recently launched James Webb Space Telescope (JWST), and would be among some of the known youngest multi-planet systems amenable for such observations.